The Sun’s magnetic field is generated by a dynamo effect, caused by convection and rotation in its envelope. It will evolve in the distant future when our star becomes a red giant, an evolved star characterised by an extended envelope and much slower rotation. The question then arises: how will the magnetic field evolve when our Sun transforms?
To answer this question, a team of researchers affiliated to the Astrophysics Department at CEA Paris-Saclay and the OSU OREME at the University of Montpellier have carried out several advanced high-performance numerical simulations of the star Pollux, a red giant that serves as an example of what the Sun could become, with a very weak magnetic field of less than 1 Gauss, due to its extended envelope.
These simulations reproduce the observed value and explain this low value by showing that between 2 and 8% of the plasma’s kinetic (motion) energy is converted into magnetic energy, depending on the size of the convection cells at the source of the dynamo. Smaller cells create less intense and more complex fields, because the correlation between magnetic and convective structures diminishes on a large scale. The study also shows that Pollux’s magnetic field reverses its polarity over several years, like the Sun’s, a phenomenon that has not yet been observed but that is suggested by the simulations and needs to be observed over a longer period to be confirmed.
In addition, this work enriches our understanding of the magnetism of giant stars and opens up new prospects for ESA’s PLATO mission, which will explore the magnetic activity of stars and its impact on exoplanets.
This study has been published in The Astrophysical Journal.
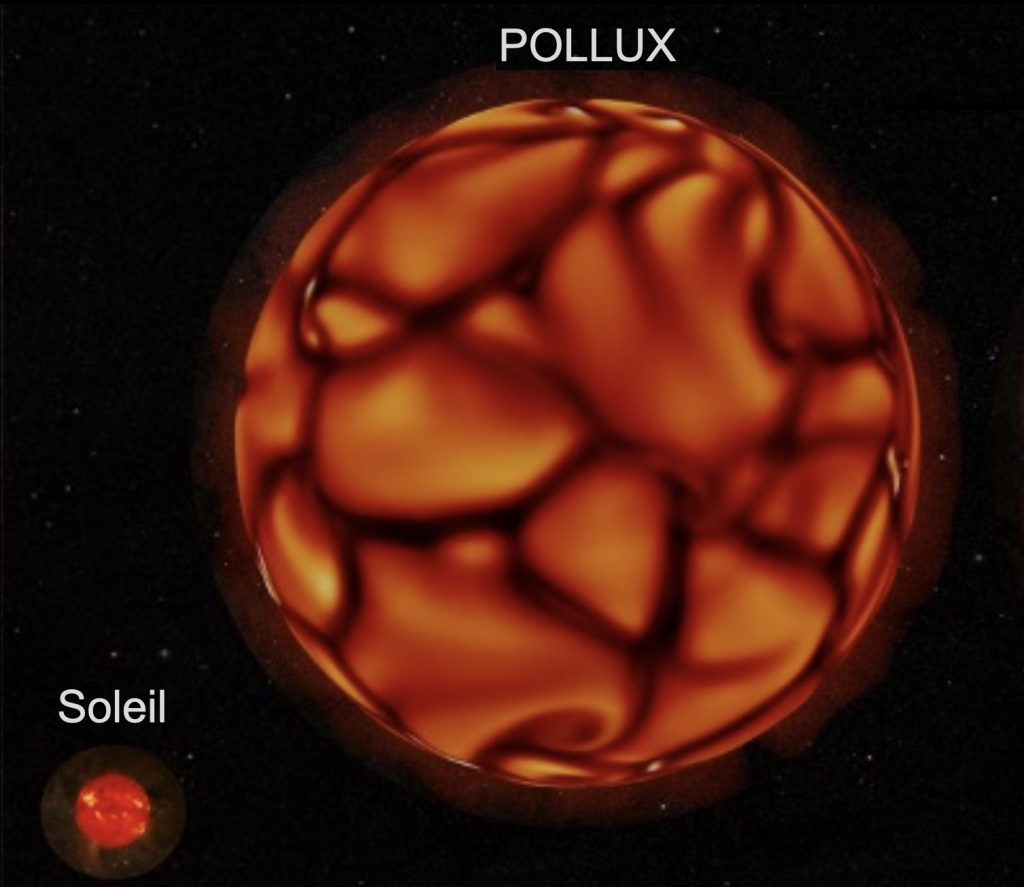
Pollux used as a proxy for the future of our Sun
The Sun is currently in the main sequence, a stable phase in which hydrogen fuses into helium in its core, producing the energy needed to maintain the star’s mechanical (hydrostatic) equilibrium. In around 5.5 billion years, the Sun will have converted all its hydrogen into helium and will enter a more unstable phase. Its core will contract until the helium begins to fuse, releasing a large amount of energy that will expand the outer layers of the star, following a thermostatic mechanism. The Sun will then become a red giant, with a diameter as large as the orbit of Venus, and a luminosity up to 2,000 times greater.
As far as the evolution of its magnetic field is concerned, things are less clear. Beyond the stable and dense radiative core of stars, the star’s plasma becomes convectively unstable, producing flows of matter on a more or less large scale. These convective cells are affected by the non-uniform (differential) rotation as a function of the radius and latitude present in the envelope, and enable a dynamo to be set up on the scale of the star, maintaining the generation of a magnetic field against its dissipation by the Joule effect. However, we do not know how this field will evolve when the Sun reaches the red giant phase, during which its envelope will expand considerably and its rotation will slow down sharply.
A team of researchers, associated with the Astrophysics Department of the CEA Paris-Saclay and the OSU OREME at the University of Montpellier, set about the task. She used the star Pollux, which has just reached the stage of red giant, as a proxy for understanding the future of our star. Pollux, which is well studied because of its proximity and luminosity, has a mass 2.5 times that of the Sun and a radius around 9 times greater (cf. Figure 1). Spectro-polarimetric observations have shown that the magnetic field at the surface is very weak, just under 1 Gauss, probably one of the weakest ever detected on a star.
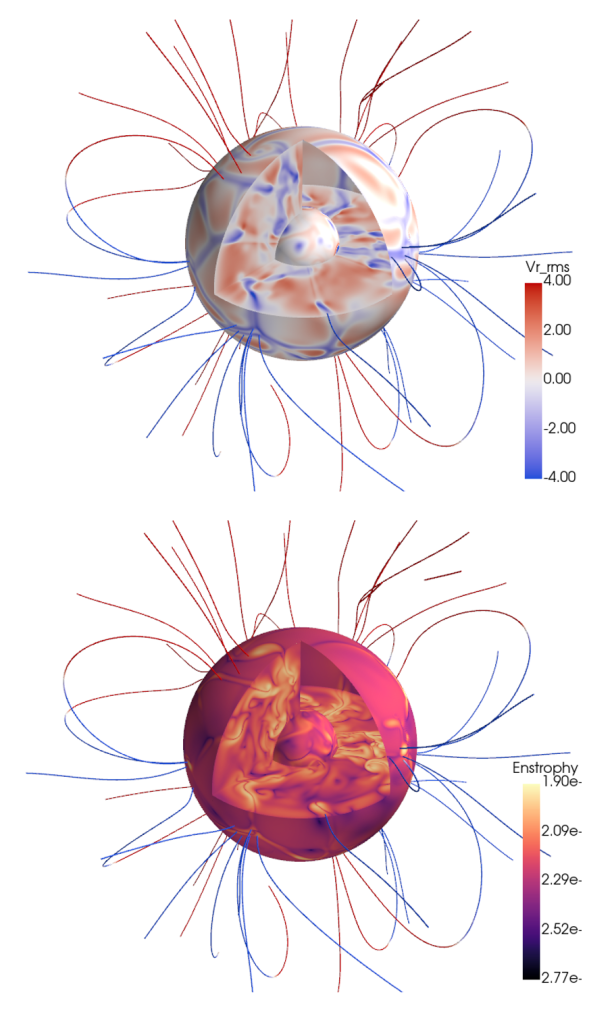
Discovery of the dynamo mechanism of giant giants, a world first
To understand the magnetic field of giants and the dynamo process in their extended convective envelope, the research team carried out a series of 3D magnetohydrodynamic simulations (Figure 2), using the GENCI supercomputers at the TGCC and Idris.
The magnetic field values deduced from the study’s numerical simulations are comparable to the very low values measured at the surface of Pollux, of the order of 1G.
The researchers also demonstrated that the geometry and intensity of this magnetic field depend directly on the size of the convection cells (see Figure 4). Small cells, illustrated by the simulation on the left in Figure 3, produce a weaker and more complex magnetic field, structured on small scales. This is explained by a less effective correlation between the convective and magnetic structures on these large scales, thus limiting the coherence and strength of the overall magnetic field as illustrated on the left of Figure 4.
Thanks to this study, researchers have estimated that between 2% and 8% of kinetic energy is converted into magnetic energy, depending on the parameters/cases considered. The discovery of such a dynamo mechanism in turbulent 3D simulations of giant stars is a world first.
Finally, as shown in Figure 4, simulations suggest that a star like Pollux could reverse the polarity of the magnetic field over periods of several years, as the Sun does. This phenomenon is not visible in the current data, which covers only about four years of observation. The study therefore predicts that regular observations over a longer period could reveal magnetic inversions at Pollux.
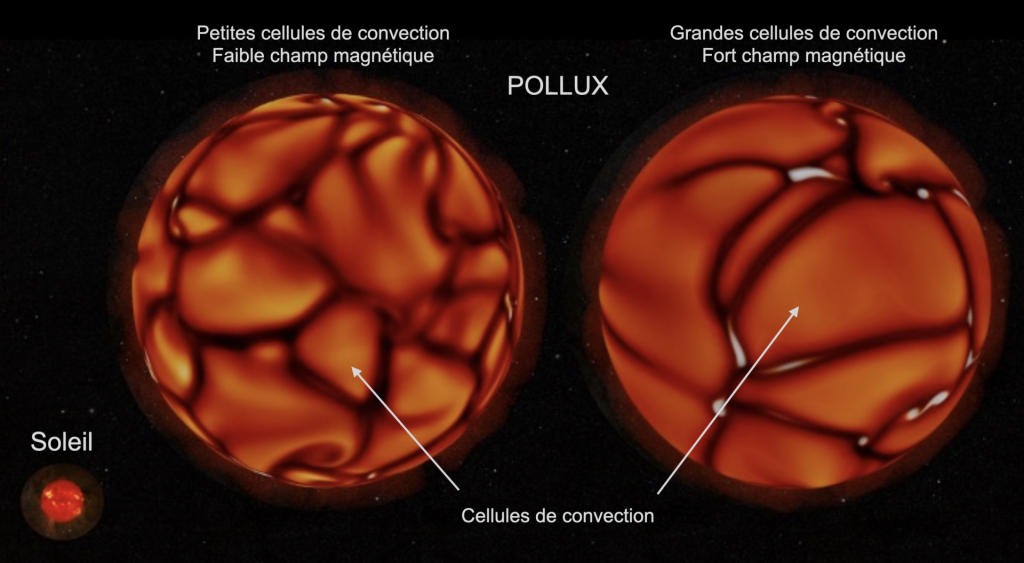
A key study for the success of future stellar and exoplanetary missions
This study of the magnetism of giant stars provides a better understanding of the stellar dynamo phenomenon by proposing a scenario in which small-scale turbulence generates a weak magnetic field in the extended envelope of these stars.
By creating magnetic activity at the surface of the star and thus causing variations in luminosity, the magnetic field complicates the detection and characterisation of exoplanets. By deepening our understanding of stellar magnetism, this research fits in perfectly with the future ESA exoplanet search mission, PLATO, to which LDE3 and LUPM are actively contributing.
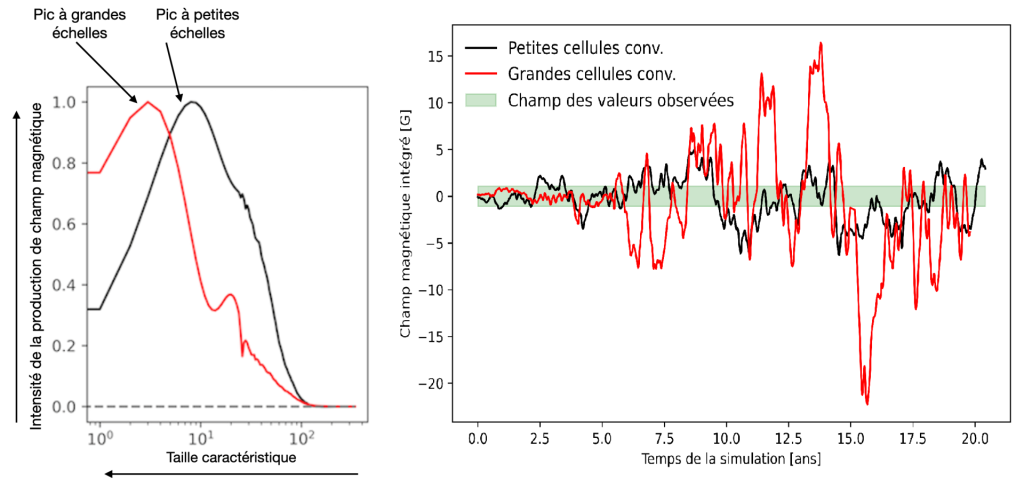
Contacts CEA-IRFU: Louis AMARD, Sacha BRUN
Publication: “Understanding Post Main Sequence Stellar Magnetism: On the origin of Pollux weak surface magnetic field”, Amard et al. 2024, The Astrophysical Journal